At its most basic form, life requires energy, water and food. Historically, we’ve depended exclusively on nature to provide those first two resources so that we could grow the third. With Scarcity Zero, this would no longer need to be the case.
An effectively unlimited supply of electricity, water and fuel gives us opportunities to revolutionize our industrial and agricultural systems, allowing us to grow produce locally in indoor farms. This produce can be healthier and of better quality than much of what we cultivate today – and more of it can be grown at greater efficiencies with shorter delivery times (and reduced environmental impact) on less land.[1]
These systems can be built close to areas where food is consumed, which reduces obstacles to transportation and delivery, especially within urban environments. Indoor farms can also be climate controlled and operate 24 hours a day, 365 days a year – dramatically increasing output and efficiency compared to traditional agricultural methods. Past obstacles to implementing them in the past stemmed primarily from material and resource costs,[2] problems that Scarcity Zero substantially reduces.
With indoor farms, as long as there is water, light and heat, the location and outside environment doesn’t matter. This allows food to be grown anywhere on the planet at any time of year, with increased yield, higher efficiency, longer growing seasons, and greater food security.
In this concept image of an indoor farming warehouse, water from storage tanks is mixed with an organic fertilizer made from excess plant matter grown within the warehouse itself. This water is then pumped through the facility and dispersed over plots of crops that grow under high-intensity lights. These crops would grow on modular wheeled platforms that can be easily moved, and the crops growing on them can be manually pollinated as necessary.
What water isn’t absorbed by crops drains into a collection mechanism in the floor, which sends the water to the bottom of the warehouse where it is filtered and placed back into circulation. As we see today, the construction of large warehouses at acceptable cost isn’t uncommon – take any Walmart, Target, Home Depot or other big-box retailer, for example. These buildings are huge, often encompassing a surface area into the hundreds of thousands of square feet. Similar structures present promising opportunities for indoor farming.
If an indoor farming warehouse had dimensions of 600' per side, that comes to a total surface area of 360,000 square feet (roughly 1/15th of the surface area of Tesla Headquarters).[3] Yet if the growing platforms were stacked, each subsequent layer adds that same surface area to the aggregate total to become a force multiplier. At five stacks, that warehouse now offers 1.8 million square feet of growing space. At ten stacks, 3.6 million. At twenty stacks, 7.2 million. Building two dozen of such warehouses, at twenty stacks each, would boast a total growing surface of 172.8 million square feet. That’s 3,967 acres - some 6.2 square miles.
As these warehouses would operate 24 hours a day, 365 days a year, their aggregate output could grow large enough to provide food for large metropolitan areas – the capabilities of which would be limited only by the number of indoor farm clusters. This setup is becoming more common, and is in use throughout many of the indoor farms that have sprung up around the world today.
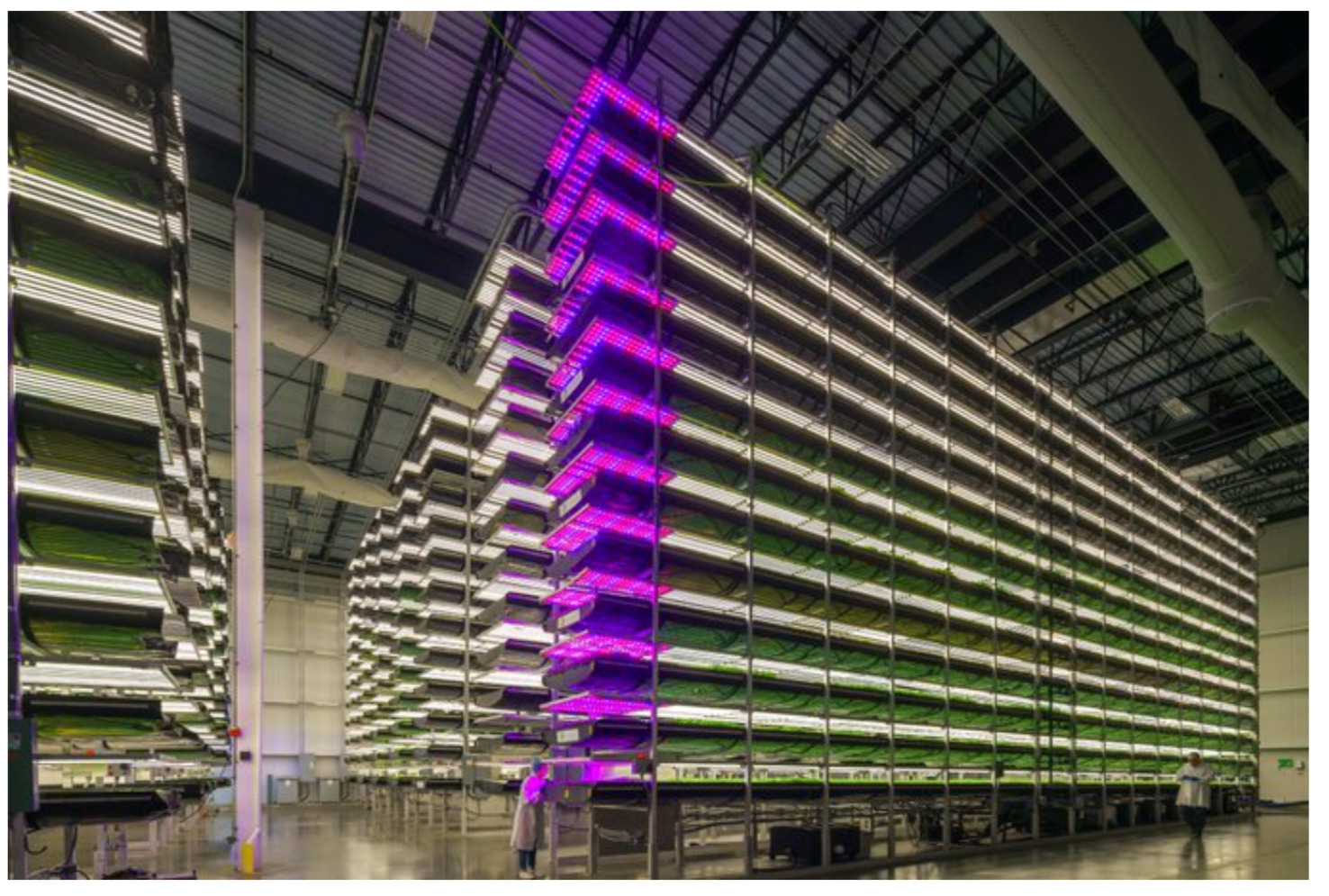
Combined, these systems create a cultivation mechanism that allows produce of effectively any kind to be grown locally. And this produce would be grown under controlled conditions – that is to say, each type of crop would be grown under ideal conditions for that type of crop. To elaborate further, here are some of the more remarkable benefits of indoor farming:
Total control of environment and constant operation. Since humans discovered how to farm, we’ve been limited to a growing season as determined by the local environment. Indoor farming completely bypasses this limitation, allowing us to emulate any growing conditions we wish. Moreover, indoor farms can be customized to the point where we’d have total control over the temperature, humidity, light spectrum, and soil composition in any given section of warehouse. And as indoor farms operate 24/7/365, they can reflect the ideal light cycle for any plant grown. This would dramatically increase overall efficiency, as there would be no seasonal slowdowns or environmental complications.
Technology-driven pest/contaminant prevention. Pests and weeds are problems in any open environment – problems we’ve tried to solve with herbicides and pesticides of varying degrees of toxicity. As they offer total control of environmental setting, indoor farms allow us to manage the presence of weeds and pests without dependence on more toxic chemicals. Examples include:
- Positive pressure. The indoor farm can be pressurized higher than the outside area, so that when a door opens, indoor air blows out of the building instead of outdoor air blowing in. Alongside worker sterilization and air filtration mechanisms, this would limit the presence of contaminants inside the farm.
- Active anti-pest measures. In the event that a pest did get in, we could respond more surgically or with more benign pesticides. We wouldn’t need to rely as strongly on more toxic treatments, because the pests they combat would be less present in the first place.
- Isolated sections. In what would also benefit food security as a whole, isolating areas of the indoor farm would hinder the ability of a pest or contaminant to spread from one area to another. This would further assist in any necessary cleanup operations should an infestation occur.
Waste management. Indoor farms can be designed to minimize the use of artificial fertilizers through composting. Whenever a plant dies, sheds material, or leaves behind waste after harvest, that material can be collected into a composting mechanism (shown in the concept image at the start of this chapter) that can be mixed with other organic fertilizers and pumped directly into the water supply used to irrigate crops. As much of the world’s soil is facing varying degrees of contamination (such as arsenic in rice and steroids in runoff water from feed lots),[4] this method translates to healthier food.
Diversity of crops. As their components would provide ideal growing environments that are naturally pest-resistant, indoor farms can encourage greater use of heirloom crops that might not fare as well as a genetically modified variant outdoors. This allows us to cultivate a greater variety of produce and shift our focus towards growing food with higher nutritional properties, expanding organic and farm-to-table markets.
Local operation. Indoor farms can be built in close proximity to metropolitan areas, so that food is grown close to the people who consume it. Food production in New York would be consumed by New Yorkers, food production in California would be consumed by Californians. This simplifies the delivery of food from farm to market, saving resources and allowing for fresher produce.
It can also present major improvements to how we provide food aid, as global anti-famine initiatives usually involve shipping food that’s already grown. With indoor farms, the system itself can comprise the aid, allowing stressed regions to grow their own produce by themselves.
Food efficiency. As touched on earlier, the benefits of indoor farming present notable benefits for efficiency. The indoor farming startup Plenty claims that indoor farms can produce roughly 350 times as much produce-per-acre of land, with only 1% of the associated water usage.[5] AeroFarms, a New Jersey indoor farming startup, claims they can produce upwards of 130 times the amount of produce grown per acre, with similar reductions in water usage.[6] Even if these numbers are inflated – which seems unlikely, considering the ideal growing conditions and 24/7/365 growing season that indoor farms provide – at even half the claimed efficiency improvements, the benefits would still be enormous compared to traditional agriculture.
In addition to growing efficiency, the efficiency of delivery, particularly as it relates to food quality, must also be considered. Approximately 35% of the fruits and vegetables we eat as Americans are imported from abroad, with leafy greens traveling an average of 2,000 miles from farm to plate.[7] Some produce travels for weeks before being served, losing, at some estimates, 45% of its nutritional value in the process.[8] Further, regardless of nutritional value, Americans rarely buy unsightly produce. Locally grown food would provide significant benefits in this area.[9]
Food security. Local food production in isolated environments also allows us to reduce security risks to our food supply. In late 2011, a Listeria outbreak in cantaloupe killed more than 20 people in the United States,[10] and food contamination (and recalls) have proven relatively frequent as of late.[11] Supermarkets across the nation are stocked with produce that comes from different states, different countries, even different continents, and it’s hard to keep track of where everything is coming from in real time. So, when an outbreak is detected, our food networks are thrown into chaos until investigators can pinpoint the source of the contamination and isolate it. With locally grown produce, security issues, however rarer, are automatically isolated since production environments are sealed.
This also protects our food supply from pathogens. Genetically modified crops, often with identical genomes, make up large swaths of our food supply – comprising approximately 90% of soy and corn.[12] Of the crops with identical genetics, any self-replicating pathogen that could infect one plant could potentially infect all of them.[13] By design, indoor farms exercise self-quarantine, which is likely the best defense they could have.
Indoor farming can provide local and sustainable food production while systematically avoiding most of the obstacles and threats that exist in agriculture today. But beyond indoor growth in warehouses, we can integrate indoor farms directly within urban environments – not only to supplement food production, but also to serve as centerpieces for more advanced cities with next-generation infrastructure.
Urban Vertical Farming
Since the end of World War II, American agriculture has increasingly represented a centralized model where the majority of food is grown in one large region (the “American Breadbasket”) and shipped elsewhere for processing and distribution. This has led to logistical challenges that indoor farms are designed to address, but providing a framework for climate-controlled indoor farming is only the first step. The next is urban vertical farming.
An urban vertical farm doesn’t use a flat plot of land or a large warehouse, but instead floors of city buildings that have been either built or modified for farming. This approach has existed in varied forms for millennia (the hanging gardens of Babylon perhaps most famous of them) and has been used in Europe since the 1850s.[14] Today, urban vertical farms are being constructed in London, Chicago, Milan and Newark, with future farms planned in several other cities.[15]
In the past, the feasibility of vertical farming has been limited by constraints inherent to resource scarcity, namely the cost of energy and materials. With the implementation of Scarcity Zero, these constraints would no longer be present in force, reducing the limitations to large-scale urban vertical farms, such as the following concepts:
At first glance, these systems might appear somewhat futuristic, but from an architectural or engineering standpoint, these designs present few challenges that have not been solved already in other industries with today’s technology.
They are well within the realm of feasibility, especially if energy costs and material limitations are removed from the equation. Accordingly, the following images show urban vertical farms that are actively operating around the world today:
Further, urban vertical farms have additional benefits that cannot be provided by warehouse-style indoor farms, making them especially attractive for cities with higher population densities. These include:
Smarter food production. While indoor farms may grow more crops at the size of a warehouse, urban vertical farms can still provide a major boost to food production. As urban vertical farms would exist directly within city centers, it effectively zeroes out the distance between production and consumption. In doing this, vertical farms redefine the notion of “farm to table,” as they would be within short distance of the millions of people they could provide food for.
Municipal water recycling. In addition to integrated water management, vertical farms could also have features that would not necessarily be available outside of an urban environment. Today, it is standard for water treatment systems to filter water based on what its purpose was. Grey water (water that’s been used for washing clothes or bathing) is treated differently than water that’s been used for cleaning dishes, and both are treated differently than water that’s used to process bodily waste. Using currently existing water filtration systems, waste water could be used in place of fresh water to grow crops in vertical farms. This would aid in recycling municipal water and also reduce the stress vertical farms could place on a given area’s water resources – unlimited water supply through the National Aqueduct notwithstanding.
This said, it’s noteworthy that food production isn’t the only application for vertical farming, as plants provide more value than just consumption. For example:
Soil is a great insulator. The idea of placing greenhouses on rooftops has been around for some time, and one of the most attractive supplementary benefits of doing so is their insulation potential. As hot air rises through a building, it escapes through the roof, which increases energy costs. Acting like a blanket, rooftop greenhouses work to keep as much energy as possible within a given building. This concept has already been applied in Italy with the Bosco Verticale towers in Milan, which were completed in 2015.[17]
Several cities have applied this concept further into law. New York City, for example, recently passed legislation requiring green roofs on new buildings within its jurisdiction.[18] Denver, Portland, San Francisco and Chicago have enacted similar measures to establish green rooftops for urban air purification and temperature control of city buildings.[19]
Plants are air purifiers. Plants are highly effective in scrubbing air of impurities and contaminants, which are often concentrated in cities. Large-scale urban agriculture through vertical farms can act as a massive, constant air filtration system. As allergies and respiratory ailments like asthma have been on the rise, this can provide a boost to public health both physically and mentally.[20]
China has taken this to heart and has begun construction of the world’s first “forest city” that will integrate plants and trees directly within city buildings.[21] Planned for a 2020 completion date, the city is expected to be covered in one million plants and forty thousand trees.[22]
Indoor parks and greener cities are socially beneficial. Regardless of how far we have come in terms of technological advancement, connection to nature is important for human happiness, lower stress levels, higher productivity, and positive life outlook. For those of us who live in cities, disconnection from nature can sow the seeds of depression, and depression is a social toxin. But could you imagine how much less stressful life would be if you could just take an elevator to the roof of your office building and hang out in a tropical park for 30 minutes on your lunch break? Or walk off a city street into a large indoor park where it was bright, colorful and warm?
Daily exposure to nature, however short, can make a major difference, which not only leads to a happier and healthier society, but also improves collective hope, and thus the collective drive to seek, build, and do greater things. And as real estate for public parks faces high costs in cities, devoting floors of a building to a welcoming public hangout can significantly lower expenses for the same deliverable – especially if the park is on the roof of a building.
Farming More Than Food
Indoor farming would significantly reduce stress on the American breadbasket, requiring outdoor farmers to grow significantly less food than they do today to meet demand. At first glance, this might seem like a trouble spot for farmers, because growing less food means making less money. So should indoor farms give them cause to worry? No – because growing less produce gives them an opportunity to grow other crops – crops that have both a higher density and commercial value than the kind that generally makes their way to supermarkets.
For example, instead of growing corn or soy, farmers could instead grow:
Algae for biofuel or plastics. The rising price of petroleum over the past 15 years – at least until the discovery of easier shale oil extraction – has led to a surge of investment in biofuels.[23] Biofuels are hydrocarbons that come from living plants, as opposed to oil that comes from fossilized plant remains. Even though Scarcity Zero would promote hydrogen as the nationwide fuel standard, biofuels and existing petroleum reserves can be devoted to a more appropriate purpose: advanced materials. Today, we use corn ethanol to make plastics, but corn is not the most effective crop we have at our disposal.
That honor goes to algae.
Several forms of algae have properties that allow for hydrocarbon production. The biofuel company Algenol claims that with today’s technology it can produce thousands of gallons of ethanol per acre of growing space, and we could increase that output with Scarcity Zero.[25] As algae has a higher land-use density than corn, a given farm that produces algae would generate more revenue than a farm dedicated to growing corn. Further, since the energy and materials industries tend to be more profitable than the food industry, what money might be lost from a move to indoor food production could then be replaced – with profits gained – from the shift to growing hydrocarbon-producing algae.
For comparison, the Department of Energy estimates that if we were to use algae to replace petroleum in all respects in the United States, we would need an area of about 15,000 square miles (roughly the size of Massachusetts and Connecticut combined).[26] That’s less than 1/7th of the space we use for corn, meaning if we were to move much of our food production indoors, we would have ample space to grow algae – and plenty of economic dividends for farmers to go along with it.
Algae for supplemental nutrition. Beyond uses in plastics and materials, algae can also be grown for added nutritional value in food products. The species chlorella, in particular, is among the most promising candidates.
By all definitions a “superfood,” dried chlorella is comprised of 45% protein, 20% lipids (fats), 20% carbohydrates, 10% vitamins/minerals and 5% fiber.[27] This composition, combined with a high photosynthetic efficiency (how well something grows in sunlight), gives chlorella one of the highest protein yields of any crop.[28] That’s why, after World War II, chlorella was considered as a solution to the then-global food crisis.[29] At the time, chlorella was difficult to grow outside of laboratories, but with advances in technology post-1950 – and the added benefits Scarcity Zero provides – we have the ability to grow a potent nutritional supplement that can be used to enhance any segment of the food supply. One that, just as importantly, can also provide supplemental nutrition in remote or isolated locations.
Alternative use of genetically modified organisms. One benefit to indoor farming is its ability to grow heirloom crops with yields that are similar to genetically modified crops in outdoor environments. However, this is not to say that genetic modification of plants is a negative thing in and of itself, but rather that its benefits can also be realized in other applications, something that bears special mention in this context.
Much of the anti-GMO movement[30] has focused on opposing any manipulation of crops at the genetic level, rather than genetic engineering that allows a plant to survive otherwise lethal pesticides and herbicides.[31] While this writing does not maintain a skeptical position on the current state of genetic engineering in our food supply, it suggests a moment of pause as to the wisdom of disavowing an entire scientific discipline because a corporation engineered plants with a genetic immunity to a relative of organophosphate nerve agents.[32]
People have been genetically engineering plants for millennia through splicing, cross breeding, and human (as opposed to natural) selection. We were modifying genomes then, we just weren’t doing it under a microscope. It’s what we do to a plant, and our guiding ethical standards when we do it, that ultimately matters. So, what if we instead extended the genetic modification of plants to a different focus? For example:
Efficiency of hydrocarbon production. Potential yields of hydrocarbon-producing algae for plastics and chemical stabilizers are already high.[33] However, we could configure the plant at a genetic level to produce even greater amounts of hydrocarbons or specific chains of hydrocarbons that can induce a higher-percent yield when producing plastics,[34] that are geared for more advanced polymerization[35] and that recycle more effectively (or biodegrade faster) than plastics today. We’ll go through this in more detail on these concepts in the next chapter.
Inclusion of bacteria. Algae isn’t the only organism that can produce hydrocarbons. Scientists in several countries have successfully modified the genetics of E. coli bacteria to produce diesel fuel that is nearly identical to the diesel derived from petroleum[36] – and, in theory, genetic modification could help us produce other hydrocarbons synthetically,[37] as well as accelerate the disposal of their plastic derivatives.[38]
Maximum growth. Beyond genetic engineering for industrial applications, food crops can be genetically modified in ways that do not raise as many concerns as the genetically modified crops of today. This might include engineering plants to maximize growth within indoor farms, produce larger and/or more nutritious products, or be able to optimally operate under a longer daylight-to-night ratio.
What’s Next?
As a system, indoor farming delivers indefinitely abundant supplies of food. Backed by the auspices of Scarcity Zero and an effectively unlimited supply of both water, electricity and fuel, it can grow enough food to feed the entire planet. This can eradicate the concept of famine as we know it and greatly improve global stability and economic growth – saying nothing of the cascading humanitarian benefits. That, by itself, is a transformational goal to reach.
Yet the tools that make it possible have a secondary, vital function through the provision of the building blocks of next-generation synthetic materials. And that, once delivered, is the final piece we need to evolve beyond a zero-sum resource paradigm, and the final piece we need to build our civilization upward to ever-greater heights.