Electricity. Fuel. Water. Food. Scarcity Zero and the systems it powers can help sustainably deliver all of these resources to effectively unlimited extents. Yet even with these four resources provided, we still need materials with which to build our ever-advancing society. Materials are themselves a resource, and as such they remain subject to the same laws of cost and scarcity as energy resources.
To address this problem, Scarcity Zero maintains additional functions intended to power systems that can synthesize and recycle sophisticated materials. With these materials, we can build things better, stronger, and less expensively than we can today. Just as importantly, these materials enable us to remove major limitations to our manufacturing capabilities, and can further revolutionize the properties and performance of what we can build. This becomes all the more beneficial once we integrate next-generation recycling and waste management technologies that become possible in a world powered by effectively unlimited energy and resources.
While many of the technologies inherent to the Scarcity Zero framework are cutting edge and future-focused, the material revolution it seeks to accelerate is very much already underway. Material science has made substantial strides over the past decade, bringing emergent synthetics to market with capabilities that would have been unthinkable until the most recent days of our modern era. Standouts in this area include nanocomposite plastics[1], high-strength polyurethane[2], ultra-high strength glass-reinforced epoxies,[3] and bleeding-edge metallurgy that's forged alloys of unprecedented strength and resilience.[4]
This effort has been amplified by parallel advances in high-performance computing - not only through the degrees of manufacturing precision it makes possible and volumes of data it can process, but further through its capability to enable sophisticated, real-time virtual modeling.[5] Consequently, material scientists are equipped with ever-advancing capabilities to rapidly assess and improve the mechanical and chemical properties of experimental materials.[6] The methods at our disposal have evolved to a point where we can routinely build synthetics that far exceed the natural capabilities of the materials that built the world as we know it today.[7] The advent of artificial intelligence, machine learning and quantum computing are all factors that stand to increase these abilities to subsequent orders.[8]
Yet Scarcity Zero presents opportunities to improve our approach to synthetic materials beyond the progress made already. Significant cost reductions in energy and critical resources are a major factor. Another is the ability to extend indoor farming to grow specialized algae that can produce next-generation biofuels and the base chemicals for high-performance plastics.[9]
The greatest opportunity, however, is found in the energy and material abundance required to mass-produce materials from latticed carbon.
Our world today is largely made from wood, stone and metal because these materials are naturally prevalent. But carbon-based synthetic materials are of a completely different class. Take graphene, for instance.
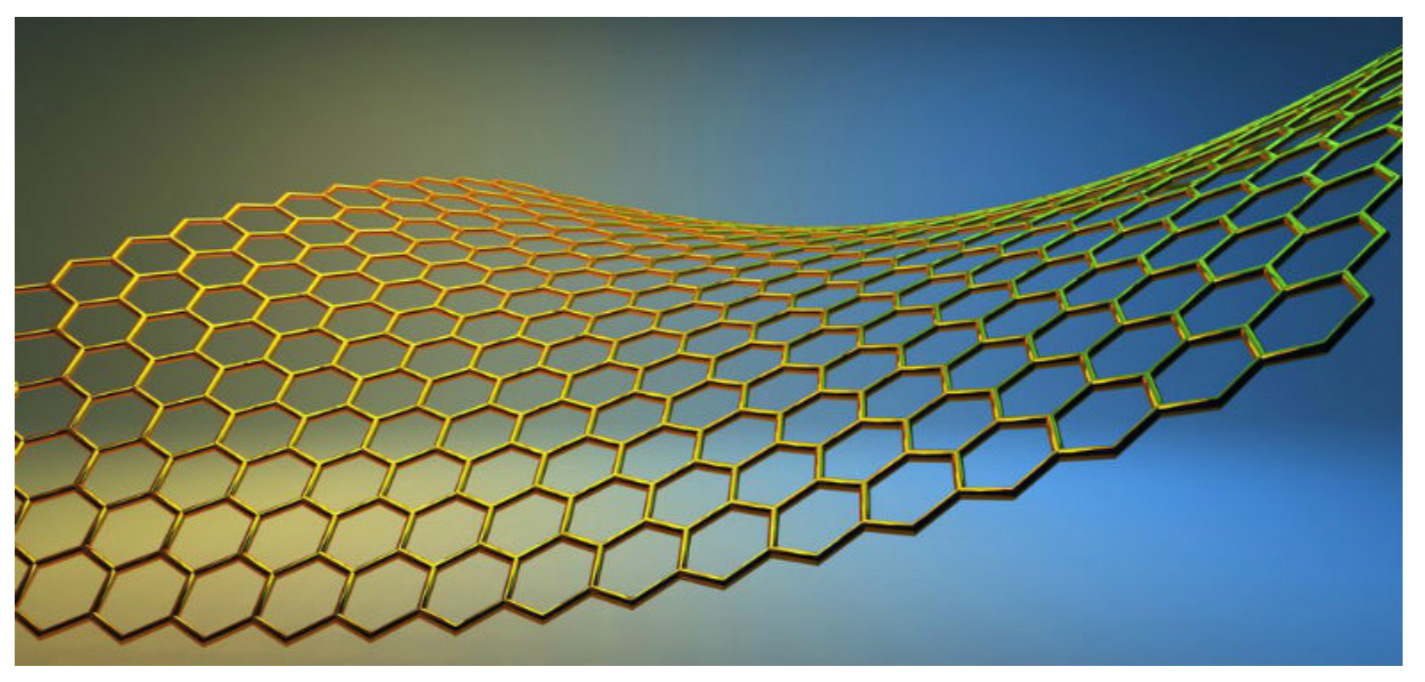
At a base concept, graphene is a ultra-thin layer of carbon (potentially as thin as one atom),[10] that takes the shape of a hexagonal lattice. Of its many capabilities, three stand out especially:
- Extreme strength. With a strength roughly 200 times that of hardened steel (20,000%), graphene is the strongest-known material on Earth – as well as one of the lightest.[11] It’s also highly resistant to both corrosion and heat, with a melting point greater than 8,132 °F (4,500 °C).
- Flexibility. As graphene is formed by thin sheets of carbon, it can retain a rigid structure or be as flexible as a sheet of plastic wrap. It can further function at full strength when assuming a range of shapes, even when designed to bend or stretch.[12]
- Conductivity. Graphene is one of the best conductors of electricity in the world.[13] This enables graphene to lend its strength and flexibility to anything electrical – as a function of either transmission, structure, or storage.[14]
The combination of these three traits makes graphene uniquely well-suited for a wide array of applications within our economic and social infrastructure. They include:
Consumer electronics. As an ultra-strong and ultra-conductive material, graphene can be used to manufacture high-performance electronics. Due to its unprecedented strength and flexibility, such electronics can withstand all but the most severe impacts. The material itself can also be used to store large volumes of data on-device.[15]
Data transfer. Graphene’s conductivity enables high capacities for data transfer, some 7,000% faster than today’s technology.[16] Researchers have conducted experiments that show graphene antennas can transmit data at speeds of up to 100 terabytes per second.[17]
Medicine. High strength and conductivity with low weight and reactivity gives graphene high potential for medical applications.[18] Potential uses include stents to prevent arterial restriction, high strength and lightweight casts for injuries and providing the physical scaffolding to help paralyzed people walk again.[19]
However, these characteristics stand second to graphene's revolutionary potential within high-performance energy storage. Graphene's characteristics enable it to function as highly efficient capacitors, which work through direct energy storage as opposed to batteries that usually require a chemical reaction to generate a charge. Most portable electricity in our day-to-day lives involves batteries, and they’re generally preferred to capacitors because they normally have a higher “energy density” – the amount of energy stored in a system by volume.[20]
But graphene’s different.
Because it’s so thin and conductive, graphene can form “supercapacitors” that can meet if not exceed the capabilities of high-performance lithium-ion batteries.[21] As graphene can be made microscopically thin, advances in manufacturing can enable us to drastically increase the potential surface area of graphene used for direct energy storage. If we were able to manufacture graphene sheets to the thickness of a micron (0.001mm), the volume of something as small as a car battery could hold more than 160,000 square feet of surface area for graphene capacitance.[22].
A 2017 paper from The Nanotechnology Journal found that graphene supercapacitors today can already deliver levels of performance that begin to eclipse industry-grade lithium-ion batteries.[23] However, even state-of-the-art lithium-ion batteries – such as those used in Tesla vehicles – are reaching their performance limits.[24] Lithium-ion batteries are also much heavier, more expensive and have increased environmental and humanitarian costs in manufacturing.[25] That graphene supercapacitors - in their infancy - can already compete with high-performance Lithium-ion batteries (as well as directly interface with hydrogen fuel cells) further supports their candidacy for future deployment as a solid-state energy solution.
So what does this mean, exactly?
Extremely strong. Completely flexible – or reliably rigid. Capable of storing tremendous energy in a tiny footprint. Graphene isn’t revolutionary because it’s impressive in each of these categories, it’s revolutionary because it’s impressive in all of these categories. Consequently, it can transform the way we build things. Consider the electric car.
Instead of an electric car storing packs of heavy batteries, the vehicle's chassis can be interwoven layers of graphene and can itself be the energy storage medium. When you think of the immense surface area that could be potentially stored within the size of a car battery[26], an entire vehicle chassis is a subsequently higher order. We could potentially reach points where we could interweave millions of square feet of energy storage medium within a single vehicle – increasing range, reliability and structural integrity at far less weight.[27]
Graphene enables us to wield strength, flexibility in form, and energy storage all at once, each potentially at a higher degree of performance than we can manage with leading commercial solutions today. Tomorrow’s skyscrapers, bridges, public infrastructure, transportation systems – each could also serve as electricity storage with revolutionary properties in terms of strength and environmental resilience. And through Scarcity Zero’s core offerings of energy, fuel and heat, we would have the means to synthesize graphene and other advanced synthetic materials easier and at less expense than we can today.